|
A Primer on Arterial Blood Gas Analysis
by Andrew M. Luks, MD(cont.)
back to Page 1 | Page 2
Step 4: Identify the compensatory process (if one is present)
In general, the primary process is followed by a compensatory process, as the body attempts to bring the pH back towards the normal range.
- If the patient has a primary respiratory acidosis (high PCO2 ) leading to acidemia: the compensatory process is a metabolic alkalosis (rise in the serum bicarbonate).
- If the patient has a primary respiratory alkalosis (low PCO2 ) leading to alkalemia: the compensatory process is a metabolic acidosis (decrease in the serum bicarbonate)
- If the patient has a primary metabolic acidosis (low bicarbonate) leading acidemia, the compensatory process is a respiratory alkalosis (low PCO2 ).
- If the patient has a primary metabolic alkalosis (high bicarbonate) leading to alkalemia, the compensatory process is a respiratory acidosis (high PCO2 )
The compensatory processes are summarized in Figure 2.
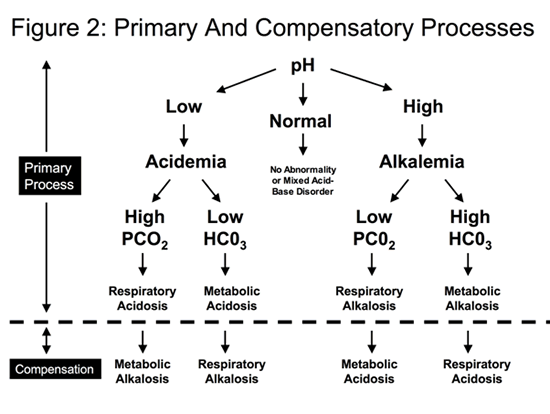
Some examples of working through this step of the process›
Important Points Regarding Compensatory Processes
There are several important points to be aware of regarding these compensatory processes:
- The body never overcompensates for the primary process. For example, if the patient develops acidemia due to a respiratory acidosis and then subsequently develops a compensatory metabolic alkalosis (a good example of this is the COPD patient with chronic carbon dioxide retention), the pH will move back towards the normal value of 7.4 but will not go to the alkalemic side of normal This might result in a pH of 7.36, for example but should not result in a pH such as 7.44 or another value on the alkalemic side of normal. If the pH appears to "over-compensate" then an additional process is at work and you will have to try and identify it. This can happen with mixed acid-base disorders, which are described further below.
- The pace of compensation varies depending on whether it is respiratory or metabolic compensation. Respiratory compensation for primary metabolic disturbance is almost immediate. For example, if someone infused hydrochloric acid through an IV and gave the patient a metabolic acidosis, the patient would rapidly begin hyperventilating and generate a respiratory alkalosis that would move the pH back towards normal. Metabolic compensation for primary respiratory abnormalities, however, is slow and may take several days. For example, if someone travels to high altitude and begins to hyperventilate due to the low oxygen levels in the atmosphere, initially there will be no metabolic compensation and they will have a high pH. Over several days, however, metabolic compensation will occur and the pH will return back towards normal.
- Despite the compensatory mechanisms, the pH may not return all the way to normal. For example, consider the following arterial blood gas in a patient with acute toluene toxicity: pH 6.95, PCO2 9, HCO3- 2. This is a primary metabolic acidosis but despite a huge degree of hyperventilation and a marked compensatory respiratory alkalosis, the pH still remains very low. In other situations (e.g., a compensated respiratory acidosis in a patient with obesity hypoventilation, or the compensated respiratory alkalosis in a pregnant woman), the pH will return closer to the normal range.
- What may appear to be a compensatory process may not actually represent true compensation. For example, consider a patient who develops an acute respiratory acidosis (large rise in the PCO2). Even though this is an acute process and renal compensation has not occured, the bicarbonate value may read 27 on the ABG. This appears elevated and would suggest metabolic compensation is starting to occur but may, in fact, not represent true compensation. How does this occur? Remember that a key relationship that governs acid-base physiology is the following:
H2O + CO2 HHCO3- H+ + HCO3-
If there is a large rise in the PCO2 , this equation will shift towards the right and the levels of bicarbonate will transiently increase. Similarly, a large fall in the PCO2 would shift the equation to the left and the bicarbonate would transiently decrease. How can you figure out whether the observed changes in bicarbonate represent true compensation or changes due to this relationship? The answer lies in another value you will see reported with the arterial blood gas—the base excess. In general, as you may recall from respiratory physiology, the base excess is defined as the difference between the patient’s HCO3-
after correction to a pH of 7.4 by a change in the PCO2 and the normal HCO3-
at pH 7.4. It can be used in the following manner to interpret changes in the HCO3-
levels.
- If the base excess is between –2 and +2 then the observed changes in bicarbonate are due to movement based on the equation above and there is no metabolic acidosis or alkalosis.
- If the base excess is less than –2, then there is a metabolic acidosis, which may be the compensatory process. Another term for this is a base deficit.
- If the base excess is greater than +2, then there is a metabolic alkalosis, which may be the compensatory process.
Be aware that if the base excess is less than -2 or greater than +2, and therefore, a metabolic process is present, the base excess value itself does not tell you whether this is a primary process or a compensatory process. It only tells you a metabolic process is present. You still need to work through the steps described here to tell whether it is the primary process or the compensatory process
- What appears as a lack of compensation may actually represent an acute process on top of a chronic process. In some cases, patients may live in a chronically compensated state of acid-base disturbance and then deviate from that state due to an acute problem. The best example is the patient with very severe COPD who might have chronic respiratory acidosis with metabolic compensation at baseline. The arterial blood gas for this patient might look like the following:
- pH 7.35
- PCO2 55
- PO2 70 HCO3- 32
Now, suppose the patient develops a severe exacerbation and comes into the ER.
At that point in time, an arterial blood gas may show
- pH 7.25
- PCO2 65
- PO2 62
- HCO3- 33
The PCO2 is now 10 mm Hg higher than before and the pH is now much further away from 7.40. If you didn’t have the initial blood gas for comparison, you would say this patient has a respiratory acidosis with only partial compensation. However, knowing the prior acid-base status, it would be more correct to say this patient has an acute-on-chronic respiratory acidosis. The acute worsening of his COPD led to a rise in the PCO2 but the bicarbonate has not risen further from baseline and the pH has not moved back towards normal because the kidneys have not had time to compensate for the acute change in the patient's condition.
Top of Page
Step 5: Determine if a Mixed Acid-Base Disorder is Present.
The 4 steps outlined above allow you to fully classify the patient’s acid-base status in the majority of cases. In such cases there is a primary abnormality and, possibly, a compensatory process. There are occasional situations, however, where patients may have more than one disorder going on at the same time. For example, it is possible for patients to have a concurrent metabolic acidosis and metabolic alkalosis or a concurrent elevated anion-gap acidosis and non-anion gap acidosis (The only combination you cannot have is a combined respiratory alkalosis and acidosis as it is impossible for the patient to hyper- and hypoventilate at the same time). These situations are referred to as mixed acid-base disorders.
One way to determine if a mixed acid-base disorder is present is to calculate the anticipated response to the primary abnormality. If the actual response deviates from this value then you know an additional process may be at work. There are equations for example, that stipulate that the bicarbonate should change by a certain amount in response to a chronic respiratory acidosis. The problem is that these equations are difficult to remember and, as a result, are tedious to apply in the acute setting. The nomograms that are often presented in respiratory physiology course are also difficult to use, as well as locate, when you need them.
An alternative approach is to calculate what is referred to as the Delta-Delta. This approach, which gives you a sense of whether the body is holding onto or losing more bicarbonate than you would expect simply based on looking at the pH, serum anion gap (SAG) and the bicarbonate values, can be done as follows:
- Calculate the Delta Gap: Measured SAG – Normal SAG (12)
- Calculate the Delta Delta: Add the Delta Gap to the measured bicarbonate (from the chemistry panel)
- Compare the Delta Delta to a normal bicarbonate (22-26):
- If the Delta Delta <22, the patient is losing bicarbonate somewhere and there is a non-gap acidosis. If you found a non-gap acidosis (either the compensatory or the primary process) in the initial steps above, then the acidosis you identify here represents the same process. If however, you did not identify an acidosis in the earlier steps or you identified a gap acidosis in the initial steps, then this last step reveals the presence of an additional non-gap acidosis.
- If the Delta Delta >26, the patient is holding onto bicarbonate and there is an additional metabolic alkalosis. If you found an alkalosis (either the compensatory or the primary process) in the initial step above, then the alkalosis you identify here represents the same process. If however, you identify an acidosis in the initial steps and then this last step reveals the presence of alkalosis, you have found an additional metabolic process.
Be aware that for these calculations to work, the chemistry panel and blood gas must have been drawn at roughly the same time. If, for example, you obtain a blood gas on a patient during a code on a patient at 6PM, you cannot use the morning chemistry panel from 6AM to do these calculations.
Examples of how to work through this last step of the process›
Top of Page
Generating Differential Diagnoses
Identifying the primary process is important because it helps you to generate differential diagnoses and decide on work-up and management. The basic differential diagnoses for the primary acid-base abnormalities are as follows:
Elevated Anion Gap Metabolic Acidosis
It is common for students and physicians to use a mnemonic to remember the common causes of an elevated anion gap acidosis. One common mnemonic is as follows:
- M: Methanol intoxication
- U: Uremia
- L: Lactic acidosis
- E: Ethylene glycol intoxication
- P: Paraldehyde intoxication
- A: Alcoholic Ketoacidosis
- K: Ketoacidosis (diabetic, starvation, alcoholic)
- S: Salicylate intoxication, Seizures, Shock
It is important to recognize that in the case of seizures and shock, it is actually the accumulation of lactic acid that is responsible for the development of the elevated anion gap acidosis.
Some people use an alternative mnemonic:
- M: Methanol intoxication
- U: Uremia
- D: Diabetic Ketoacidosis
- P: Paraldehyde intoxication
- I: Infection (sepsis)
- L: Lactic acidosis
- E: Ethylene glycol intoxication
- S: Salicylate intoxication, Seizures, Shock
Other diagnoses that don’t fit in these mnemonics can also cause an elevated anion gap acidosis and should be considered in cases where your initial work-up does not identify an underlying cause. These other diagnoses would include:
- Cyanide or carbon monoxide poisoning
- Excess inhaled beta-agonists
- Hereditary disorders (eg. glucose-6-phosphatase deficiency)
- D-Lactic acidosis (jejuno-ilea bypass, small bowel resection)
- Medications (iron, isoniazid, zidovudine)
- Toluene intoxication
- Massive Rhabdomyolysis
As noted above, in several of these cases (eg. carbon monoxide poisoning, excess beta-agonists), the elevated anion gap derives from accumulation of lactate.
You should also be aware that there is a differential diagnosis for a low serum anion gap. It includes the following items:
- Fall in unmeasured anions (eg. Hypoalbuminemia)
- Increased unmeasured cations (hyperkalemia, hypercalcemia, hypermagnesemia)
- Lithium
- Multiple Myeloma
- Bromide (found in Pyridostigmine Bromide used in the treatment of myasthenia gravis and some herbal medications)
Normal Anion Gap Metabolic Acidosis (non-gap acidosis)
The differential diagnosis includes:
- Gastrointestinal bicarbonate losses:
- Diarrhea
- Ureteral Diversion (ileal loop)
- Renal bicarbonate losses:
- Carbonic anhydrase inhibitors (eg. acetazolamide)
- Renal tubular acidosis
- Aldosterone inhibitors or hypoaldosteronism
If the cause of the non-gap acidosis is not clear based on the patient history, you can identify whether the problem is renal or gastrointestinal losses by calculating a urine anion gap:
Urine Anion Gap (UAG) = (Urine Na+ + Urine K+) – Urine Cl-
A positive value (UAG >0) suggests the metabolic acidosis is due to a renal etiology, whereas a negative value (UAG <0) points to a gastrointestinal source.
Metabolic Alkalosis
The differential diagnosis includes:
- Chloride Responsive Alkaloses:
- - Vomiting
- - Nasogastric suction
- - Diuretics
- Chloride Unresponsive Alkaloses:
- - Hyperaldosteronism
- - Cushing’s syndrome
- - Licorice ingestion
- Bartter’s syndrome
- Excess alkali intake (e.g., milk alkali syndrome)
If the cause of the metabolic alkalosis is not clear based on the patient history, you can obtain a urine chloride level to help determine the cause. If the urine chloride level is <15 the patient has a "chloride responsive alkalosis" which can be corrected with saline (NaCl) administration. This typically happens with gastrointestinal losses or intravascular volume depletion (i.e., a contraction alkalosis with diuretic use). If the urine chloride is >15, the patient has a "Chloride Unresponsive Alkalosis."
Respiratory Acidosis
A primary respiratory acidosis implies that the patient is hypoventilating or not ventilating enough in the face of high CO2 production. This can be seen in the following settings:
- Acute intoxication with narcotics or other sedative medications
- Severe metabolic encephalopathy
- Obesity hypoventilation
- Severe chronic obstructive pulmonary disease
- Acute upper airway obstruction
- Neuromuscular disorders (e.g., Guillan Barre, Myasthenia Gravis, Botulism, Amyotrophic Lateral Sclerosis)
- Later stages of a severe asthma exacerbation (e.g., the patient is tiring out)
- Thoracic cage trauma (flail chest)
- Inappropriately low minute ventilation settings on mechanical ventilation
Respiratory Alkalosis
A primary respiratory alkalosis implies that the patient is hyperventilating. This can be seen in the following settings:
- Early stages of an asthma exacerbation
- Anxiety attack
- Acute hypoxia (hypoxic ventilatory response)
- Pregnancy or other cases of elevated progesterone
- Cirrhosis and/or hepatic encephalopathy
- Salicylate intoxication
- Central nervous system disease
Top of Page
Use Old Arterial Blood Gas Results to Your Advantage
There are certain clinical situations where a review of the patient’s prior arterial blood gas results can be of great value (if the patient did, in fact, have a sample drawn in the past). To see how prior ABG results may be of use, consider the following scenario.
A patient with very severe COPD was found ashen and pulseless on the wards and a Code 199 was called. The initial arterial blood gas revealed
- pH 7.32
- PCO2 50
- PO2 105
- HCO3- 25
- on an FIO2 of 1.0.
This patient was acidemic with a high PCO2 and a normal bicarbonate and it was determined that he had a primary respiratory acidosis without metabolic compensation. That is the correct interpretation if all you have is the information from that one arterial blood gas. However, the patient did have an earlier arterial blood gas, well before his cardiac arrest whose results were as follows:
- pH 7.38
- PCO2 80
- PO2 72
- HCO3- 48
These results are consistent with a primary respiratory acidosis and a compensatory metabolic alkalosis that reflects his history of very severe COPD and known carbon dioxide retention. Of note, his baseline bicarbonate is 48, well higher than the "normal" appearing value of 25 that was obtained during the code. If all you did in this situation were look at the ABG drawn during the code, you would see a "normal" bicarbonate and might not realize that he has developed a severe metabolic acidosis. If, however, you have his old blood gas values, you would correctly note that his bicarbonate has fallen dramatically. As a result, he likely has some problem (e.,g. sepsis) that caused a severe metabolic acidosis and ultimately led to his decompensation.
Top of Page
Oxygenation
The arterial blood gas also provides information about the oxygenation status of the patient. In general, there are two basic pieces of information you can obtain using the PO2 on a blood gas: insight into the cause of the hypoxemia and an assessment of the adequacy of gas exchange.
Assessing the Cause of Hypoxemia
There are five broad categories of problems that cause hypoxemia:
- Low inspired partial pressure of oxygen (eg. high altitude)
- Hypoventilation
- Shunt
- Low ventilation relative to perfusion (low V/Q)
- Diffusion limitation (rarely an issue at sea-level)
When a patient presents with unexplained hypoxemia, you can begin to sort through the differential diagnosis by calculating the alveolar-arterial oxygen difference (AaO2 Difference). You begin this calculation by determining the alveolar PO2 using the alveolar gas equation:
PaO2 = (PB – 47) FiO2 – PaCO2 /R
Whereby PB = barometric pressure, FiO2 = the inspired oxygen concentration and R = the respiratory quotient. If you have trouble remembering the full equation, you can simplify things by remembering the PIO2 of room air where you live. The PIO2 is the first term of the alveolar gas equation (PO2 = [PB – 47] FiO2). For example, the PIO2 while breathing ambient air at sea level is 150 mmHg whereas if you live in Boise, Idaho, where the average PB is 695 mmHg, the PIO2 of room air is 135 mmHg.
Once you have calculated this value, the AaO2 Difference is calculated as follows:
AaO2D = PAO2 – PaO2
The normal value for the AaO2 Difference is about 10-15 mm Hg. If you determine that the patient has a normal AaO2 Difference, then the hypoxemia can be attributed to hypoventilation or a low inspired partial pressure of oxygen. If, however, you find that the patient has an elevated AaO2 difference then they have some process going on that is causing shunt or low V/Q, such as pneumonia, that is contributing to the observed hypoxemia.
There are a few important points to be aware of regarding the use of the AaO2 Difference.
- In order to use the alveolar gas equation you must have an accurate assessment of the FiO2. The FiO2 will be accurate when your patient is on room air, non-invasive or invasive mechanical ventilation or is on a high-flow facemask system. When patients are on supplemental oxygen using nasal cannula, regular face masks, Venturi masks or non-rebreather masks, the FiO2 is not reliable; these systems often deliver less gas flow than the patient is requiring for their minute ventilation and, as a result, they end up entraining in a lot of room air which dilutes out the intended inspired oxygen concentration.
- The "normal" AaO2 Difference increases with age. Some sources state that the upper limit of normal for the AaO2 Difference is equal to: 2.5 + (0.21 x age).
- The "normal" AaO2 Difference varies based on the FiO2. On an FiO2 of 1.0, the normal AaO2 difference is 100 mmHg. Also, if you go to high altitude, where the barometric pressure is decreased and the PIO2 falls, the normal AaO2 Difference is lower than 10.
- Make sure you are using the correct value for R. In general, we use a value of 0.8. However, if your patient is on an FiO2 of 1.0, then R is taken to be 1.
Top of Page
Assessing the Adequacy of Gas Exchange
Suppose your patient has PaO2 of 85 that results in an SpO2 around 97-98%. Even though these values are adequate for sustaining the patient, they do not mean that gas exchange is normal. In fact, a patient can have a PaO2 and SpO2 in this range and still have markedly abnormal gas exchange. As a result, whenever you interpret the PaO2 on an arterial blood gas, you must look at that number in light of the amount of oxygen that is being delivered to the patient. There are several ways you can get a sense of this value:
The P/F Ratio
This is the ratio of the PaO2 (in mmHg) to the FiO2 (expressed as a decimal). The smaller the value, the worse the patient’s gas exchange. For example, consider a patient with a PaO2 of 80. If they are on an FiO2 of 0.3, the P/F ratio is 266. If they are, instead, on FiO2 of 0.8, the P/F ratio is only 100, a much worse value that signifies greater impairment in gas exchange. The P/F ratio is commonly used in patients on mechanical ventilation as part of the assessment of whether they have the Acute Respiratory Distress Syndrome (ARDS). A P/F ratio below 300 is consistent with Acute Lung Injury, while a P/F ratio below 200 is consistent with ARDS (provided the patient meets the other criteria including a CXR with diffuse bilateral opacities and evidence that cardiac function is normal).
The AaO2 Difference
The same number that is used to help determine the cause of hypoxemia can also be used to determine the adequacy of gas exchange. In patients with an elevated AaO2 Difference, larger values imply a greater degree of either low V/Q areas and/or shunt. The size of the AaO2 Difference does not help you distinguish between low V/Q and shunt, however. The way to make that determination is to measure the response to supplemental oxygen administration. The PaO2 will rise and the AaO2 difference will shrink in patients with low V/Q as the primary cause of their hypoxemia (e.g., the COPD patient during an exacerbation) whereas in shunt, supplemental oxygen does not lead to improvements in the PaO2.
Both methods for assessing the adequacy of gas exchange require an accurate FiO2 measurement, which, as noted above, can only be assured if the patient is on room air, a high flow face mask, non-invasive mechanical ventilation or invasive mechanical ventilation.
Top of Page
Special Situations To Be Aware of In Evaluating the PaO2 on an ABG
There are a few special situations of which you should be aware when assessing the PaO2 on an arterial blood gas.
The Hypothermic Patient
Hypothermia can affect the accuracy of the PaO2 measurement by causing changes in the position of the Hb-O2 dissociation curve. If you do not inform the laboratory of the patient’s body temperature at the time the sample was measured, you may measure an artificially high PaO2. To understand how this happens, consider the following scenario. Suppose you see a patient in the emergency room with a temperature of 32°C and draw an ABG while he is still at that temperature. At this point in time, his Hb-O2 dissociation curve has shifted to the left and, as a result, for any given oxygen content of the arterial blood, the PaO2 is lower than it would be at a normal temperature. When the sample is sent to the lab and placed in the blood gas analyzer, however, the machine will warm the sample to 37°C before doing the measurement. This warming process shifts the Hb-O2 dissociation curve back to the right. Because the oxygen content of the blood has not changed, this rightward shift will lead to a higher measured PaO2 than if the measurement had taken place without warming the blood. For this reason, you should always notify the lab about the patient’s temperature at the time of the measurement if they are hypothermic so they can make the appropriate adjustments in the blood gas analyzer.
Leukocyte Larceny
This is a rare situation that may be seen when working on an oncology service with patients presenting with acute leukemia or other patients with extremely high white blood cell counts. White blood cells are metabolically active and consume oxygen. In the time between when a sample is drawn from the wrist and the measurement is made in the blood gas analyzer, the white blood cells may consume enough oxygen to decrease the PaO2 from the actual value at the time the sample was drawn. The net result is you get a PaO2 result that is much lower than what you would expect based on the oxygen saturation at the time the sample was drawn. This situation, which may be seen in leukemic patients with WBC counts of 50,000 or greater, can occur even if the sample is cooled immediately and analyzed within 10 minutes. This problem can be avoided by putting the sample on ice and adding potassium cyanide to halt oxygen consumption.
Be aware that there are other situations in which you will see a discrepancy between a high SpO2 and a low PaO2 on the ABG. These include:
- Spuriously high SpO2value
- Inadvertent venous blood sampling
- Excessive time delay in sample analysis
- Air bubbles left in the blood gas sample
Methemoglobinemia
In leukocyte larceny, the PaO2 is lower than you would expect given the SpO2. In methemoglobinemia, the opposite occurs; the patient has a low SpO2 (typically in the mid-upper 80% range) but the PaO2 is much higher than you would expect from that value. In this case, the PaO2 is actually a valid reading and the discrepancy reflects important pathology.
In this situation, there is some insult (usually a medication such as dapsone) that leads to oxidation of the iron in the hemoglobin molecule from the 2+ valence to a 3+ valence. As a result, the hemoglobin molecule changes conformation and does not bind oxygen adequately. Blood with the altered hemoglobin molecule has different light absorption properties that lead you to measure a lower saturation using a pulse oximeter. Under normal circumstances, when the PaO2 is high, you expect to see a very high saturation. However, in methemoglobinemia, even though you may have the patient on a lot of supplemental oxygen and the PaO2 is very high, the oxygen will not bind to hemoglobin and, as a result, the saturation will continue to be low (Because of the absorption properties of methemoglobin, the saturation typically remains around 87-88%).
The tip-off to this diagnosis comes when you see a patient with a low saturation that remains low despite putting them on supplemental oxygen, but find a high PaO2 on their blood gas. If you see this pattern and suspect the diagnosis, the next step is to ask the laboratory to check a methemoglobin level on the blood gas sample.
Once the diagnosis is confirmed, you must then identify and stop the offending agent and, in some case, initiate disease-specific therapies. A useful mnemonic for drugs that have the potential to cause methemoglobinemia is:
- L: Lidocaine (and other "-caine" local anesthetics)
- A: Anti-malarials
- N: Nitrites/Nitrates
- D: Dapsone (perhaps the most common offender)
back to Page 1 | Page 2
*Software capable of displaying a PDF is required for viewing or printing. You
may download a free copy of Adobe Reader from the Adobe
website.
Top of Page
|