A Primer on Mechanical Ventilation
by David J Pierson MD
Print primer [220k PDF*]
Introduction
Although mechanical ventilation is a key component of intensive care, unfamiliar jargon and technical detail render it confusing and formidably difficult for many clinicians. The rapidity and complexity of change in this area of respiratory medicine in recent years adds to the problem. Most of the current literature and nearly all the controversy in mechanical ventilation apply to only a small fraction of the patients who are intubated and ventilated in acute care hospitals. This small fraction consists of those with severe respiratory failure due to acute diffuse lung injury—acute lung injury (ALI) or the acute respiratory distress syndrome (ARDS)—and also those with severe obstructive lung disease (COPD or asthma). For the other 80 or 90 percent of ventilated patients the issues are much less difficult.
At best, mechanical ventilation can support gas exchange and lung inflation in a reasonably normal fashion. It is supportive, replacement therapy only; there is no disease that intubating and ventilating a patient will cure. When done as well as we know how, mechanical ventilation can come close to replacing the normal functions of the lungs and chest bellows. Considering the many adverse consequences of intubation and positive-pressure ventilation, the clinician should always be striving to make the period of ventilatory support as short as possible.
This primer summarizes the main issues in mechanical ventilation, explains many of the modes, settings, and terms involved, and reviews aspects of monitoring and complications with which the clinician should be familiar. It focuses on invasive mechanical ventilation (that is, on ventilating intubated patients), and on the care of adults rather than children or infants. Parts of it are adapted from the chapter on invasive mechanical ventilation in Clinical Respiratory Medicine, by Albert, Spiro, and Jett [ref-1].
Table 1[27k PDF*] lists a number of factors that are key to developing a rational approach to mechanical ventilation in acute illness. It is also important that the fundamental goals and objectives of this therapy (Table 2 [28k PDF*]) be understood, both in general and relative to the individual patient.
Top of Page
Indications for Mechanical Ventilation
In the absence of a contraindication, mechanical ventilation is indicated whenever any of the circumstances listed under "clinical objectives" in Table 2 [28k PDF*] exists to a degree that threatens the life of the patient. Apart from apnea, however, few individual symptoms, signs, or laboratory findings by themselves always mandate the initiation of ventilatory support. Rather, this therapy becomes necessary in the presence of the right combination of clinical setting, severity of abnormality, and rapidity of development or worsening of physiologic abnormality. A summary of the main categories of indications for invasive ventilatory support is provided in Table 3 [31k PDF*].
Apnea and Impending Respiratory Arrest
No one would argue with providing ventilatory support for a patient who has stopped breathing altogether. On the other hand, "impending respiratory arrest" is difficult to define prospectively, and attempts to study it as an indication for intubation and mechanical ventilation have so far proved unsuccessful. These things are commonly carried out because the clinician judges the patient to be in severe respiratory distress, "tiring," or "about to arrest," but inter-observer variation and the extent to which these subjective impressions predict an unfavorable outcome if intubation is not performed have not been investigated.
Exacerbation of Chronic Obstructive Pulmonary Disease
The substantial number of studies of noninvasive positive-pressure ventilation (NPPV) in severe exacerbations of COPD has not directly addressed the validity of the criteria used for intubation. The writing committee for the Global Initiative for Obstructive Lung Disease (www.goldcopd.org), using best available evidence, recommends invasive mechanical ventilation when patients with acute exacerbations of COPD have cardiovascular instability, somnolence or other altered mental state, uncooperativeness, a high risk of aspiration, copious or very viscous respiratory tract secretions, any craniofacial condition (such as recent trauma or surgery) potentially rendering NPPV difficult, or extreme obesity. Very severe or progressive respiratory acidosis is also accepted as an indication, but agreement is lacking as to whether a pH of 7.25, a PaCO2 of 60 mm Hg, or some other thresholds should be used.
Acute Severe Asthma
Retrospective studies have shown that relatively few patients with acute severe asthma require invasive mechanical ventilation, but no clinical trials to define the specific indications have been reported. These indications may be similar to those for acute COPD exacerbations, although the potential for more rapid physiologic improvement in asthma, and the fact that patients with asthma are typically younger and healthier than those with severe COPD raise doubt about this assumption. Unlike the situation with COPD exacerbations, the benefit of NPPV in acute severe asthma has not yet been clearly established.
Neuromuscular Disease
In acute respiratory insufficiency complicating neuromuscular disorders such as the Guillain-Barré syndrome and myasthenia gravis, there is agreement among experienced clinicians that invasive mechanical ventilation is best initiated before the patient develops frank respiratory acidosis. Although vital capacity and the maximum inspiratory pressure generated against an occluded airway have been used in assessing the need for intubation in such patients, the thresholds shown in Table 3 have not been established through prospective studies.
Acute Hypoxemic Respiratory Failure
Severe hypoxemia by itself is seldom an indication for invasive mechanical ventilation. For example, isolated hypoxemia in patients who have diffuse pneumonia or pulmonary edema can often be managed with high-flow oxygen by mask, with or without continuous positive airway pressure (CPAP). Typically, patients who demonstrate severe hypoxemia in the setting of severe acute illness have other indications for ventilatory support, such as evidence for excessive work of breathing or diminished ventilatory drive. There is no evidence to indicate what threshold of PaCO2/FIO2 or other measure of oxygenation failure should be used as an independent indication for intubation and mechanical ventilation, or in fact whether such a threshold exists.
Several studies of NPPV in various forms of acute hypoxemic respiratory failure have yielded inconclusive results, although available data suggest that it may be possible to avoid intubation in some immunocompromised patients with the use of this modality. The presence of cardiovascular instability, altered mental status, or evidence of the inability to adequately protect the lower airway are clear indications for intubation in acute hypoxemic respiratory failure; in the absence of these findings it may be reasonable to attempt NPPV if there is single-organ failure in an otherwise healthy patient and reason to believe that the patient's condition will improve rapidly.
Heart Failure and Cardiogenic Shock
Available evidence on the management of cardiac pulmonary edema indicates that CPAP or NPPV may improve gas exchange--possibly with a reduced need for intubation--but that rates of clinical recovery and other outcomes may not be different compared to management without these interventions. Cardiogenic shock, however, may represent a separate indication for invasive mechanical ventilation, to decrease the oxygen cost of breathing at a time of severely impaired cardiac function. While this has not been subjected to a prospective clinical trial, retrospective studies of patients managed with intra-aortic balloon pumps have found higher rates of weaning from the pump and improved hospital mortality in patients who were intubated and ventilated.
Acute Brain Injury
Short-term hyperventilation can rapidly decrease intracranial pressure in patients with traumatic brain injury by constricting cerebral blood vessels and decreasing both cerebral blood flow and cerebral blood volume. However, available evidence indicates that routine hyperventilation in such patients does not improve survival or neurologic outcome, and may in fact worsen the latter. Although brief periods of hyperventilation are used acutely to reduce sudden increases in intracranial pressure while more definitive measures are undertaken, the presence of acute brain injury is not by itself an indication for hyperventilation.
Top of Page
Contraindications to Invasive Mechanical Ventilation
In general, intubation and mechanical ventilations should not be used in the following circumstances:
- No indication for ventilatory support exists (see Table 3 [31k PDF*])
- Noninvasive ventilation is indicated in preference to invasive mechanical ventilation
- Intubation and mechanical ventilation are contrary to the patient’s expressed wishes
- Life-support interventions, including mechanical ventilation, would constitute medically futile therapy
Top of Page
Types and modes of mechanical ventilation
A confusing assortment of possible ways to mechanically ventilate a patient’s lungs is available to the clinician, and these ways are distinguished by numerous variables. Phase variables are used to initiate one of the three phases (trigger, limit, and cycle) of the ventilatory cycle.
- The trigger variable, which causes inspiration to begin, can be a preset pressure variation (pressure triggering), a preset volume (volume triggering), a designated flow change (flow triggering), or an elapsed time (time triggering).
- The limit variable is the pressure, volume, or flow target that cannot be exceeded during inspiration. An inspiration may thus be limited when a preset peak airway pressure is reached (pressure limiting), when a preset volume is delivered (volume limiting), or when a preset peak flow is attained (flow limiting).
- Cycling refers to the factors that terminate inspiration. A breath may be pressure, volume, or time cycled when a preset pressure, volume, or flow as time interval has been reached, respectively.
Three different types of breath can be provided during mechanical ventilation, depending upon whether the ventilator or the patient does the work and whether the ventilator or the patient initiates (triggers) the breath. These types are mandatory, assisted, and spontaneous breaths.
- Mandatory breaths are machine cycled, and are triggered, limited, and cycled by the ventilator. The patient is entirely passive, and the ventilator performs the work of breathing.
- Assisted breaths are like mandatory breaths in that they are limited and cycled by the ventilator, but are triggered by the patient. Breathing work is thus partly provided by the ventilator and partly by the patient.
- Spontaneous breaths are triggered, limited, and cycled by the patient, who performs all the work of breathing.
The relationship between the various possible types of breath and the inspiratory phase variables just discussed is called a mode of ventilation. Table 4 [30k PDF*] lists and describes the modes currently available for positive-pressure ventilatory support.
The distinction between volume-targeted and pressure-targeted ventilation is clinically important. Volume-targeted modes deliver a fixed tidal volume (Vt) with each breath. This means that airway pressure during a given breath can vary depending on the resistance to airflow during inspiration and on the patient’s lung and chest wall compliance.
Fig 1
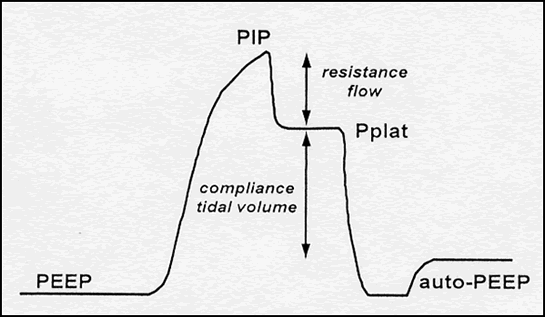
Figure 1: Pressure profile of a volume-targeted breath in which flow is interrupted at end-inspiration, allowing measurement of the quasi-static properties of the respiratory system. PEEP, positive end-expiratory pressure; PIP, peak inspiratory pressure; Pplat, end-inspiratory plateau pressure; auto-PEEP, pressure above the set level of PEEP that is generated by dynamic hyperinflation due to incomplete exhalation in the presence of obstructive lung disease.
As shown in Figure 1, the pressure profile of a volume-targeted breath has several components. Once the breath has been delivered, an end-inspiratory hold maneuver can be performed to measure the static or plateau pressure (Pplat). The latter is a reflection of lung distention and is used to calculate the static compliance of the respiratory system (equals DV/DP). During inspiration, airway pressure reflects the resistance to flow as well as the compliance of the system. Thus, an increase in inspiratory flow, bronchospasm, or airway secretions increases PIP, but does not directly affect Pplat.
Volume-Targeted Modes
The most commonly used volume-targeted ventilator modes are assist/control (A/C, or AMV) and synchronized intermittent mandatory ventilation (SIMV). Figures 2-4 illustrate these schematically along with controlled mechanical ventilation (CMV), conceptually the “default mode” of volume-targeted ventilation. With CMV, the patient receives a preset number of fixed-volume breaths and cannot increase minute ventilation by triggering more machine breaths or by breathing spontaneously between them. The difference between A/C ventilation and CMV is that the patient may trigger additional fixed-volume machine breaths if desired. With intermittent mandatory ventilation (IMV), a fixed number of preset-volume breaths is delivered by the ventilator and, if desired (and capable), the patient can also breathe spontaneously from the ventilator circuit. Conceptually, IMV is therefore somewhat like being on both CMV and a T-piece at the same time. The theoretic risk of ‘stacking’ a mandatory breath on top of a large spontaneous breath, which might produce barotrauma, means that most ventilators deliver IMV in such a way that mandatory breaths can only be delivered after expiration is sensed, called SIMV.
Fig 2: controlled mechanical ventilation (CMV)
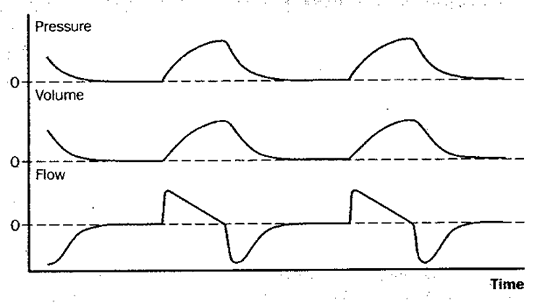
Figure 2: Schematic depiction of changes in pressure at the airway opening, lung volume, and flow during controlled mechanical ventilation (CMV). With CMV, all breaths are machine-triggered mandatory breaths, and the patient is passive throughout the cycle.
Fig 3: assist/control ventilation (A/C, or AMV)
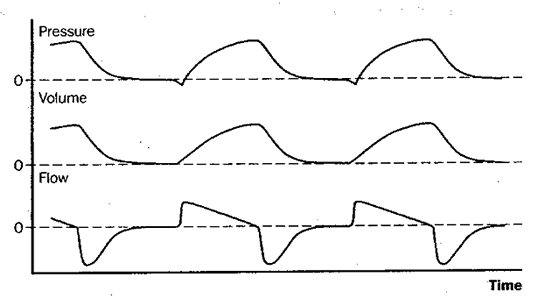
Figure 3: Schematic depiction of changes in pressure at the airway opening, lung volume, and flow during assist/control mechanical ventilation (A/C, or AMV). Assist/control is essentially the same as CMV except that the patient may, if desired, trigger the set-volume machine breaths at a more rapid rate. Note on the pressure tracing that in this example each breath is patient-triggered, as shown by the slight negative deflection of the airway pressure tracing as it is initiated.
Fig 4: synchronized intermittent mandatory ventilation (SIMV)
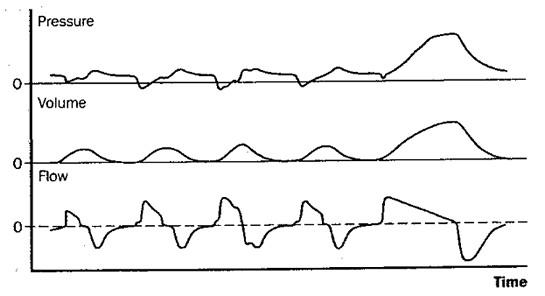
Figure 4: Schematic depiction of changes in pressure at the airway opening, lung volume, and flow during synchronized intermittent mandatory ventilation (SIMV). With SIMV, a set number of machine-triggered, mandatory breaths are delivered, as with CMV. However, in SIMV the patient can also breathe spontaneously between mandatory breaths if desired. When the mandatory rate in SIMV is sufficient to provide all the ventilation the patient needs, this mode is effectively the same as CMV.
Full ventilatory support is provided by CMV, which means that all work performed on the respiratory system during ventilation is provided by the ventilator (the patient is passive). In A/C ventilation, full ventilatory support is provided when the patient is not triggering, but partial ventilatory support when the patient breathes at a rate greater than the fixed backup rate. Studies have shown that patient work can be substantial in A/C, continuing throughout the inspiratory phase, particularly if the patient is air-hungry and the inspiratory flows provided by the ventilator are low. In SIMV, full ventilatory support is provided when the patient is not attempting to breathe above the mandatory rate, and partial ventilatory support when any spontaneous ventilation is present.
Pressure-Targeted Modes
A number of modes are available that preset the maximum inflation pressure rather than a fixed Vt. Most widely used among these are pressure support ventilation (PSV) and pressure control ventilation (PCV), illustrated in Figures 5 and 6.
With PSV, the patient breathes spontaneously and is assisted with every breath to a preset inspiratory pressure target. Although the technical aspects of its delivery are different, this is conceptually the same as intermittent positive-pressure breathing (IPPB), used in the past as a means of ventilatory support but now primarily an adjunct to lung-expansion maneuvers for preventing or treating atelectasis. Pressure support can be combined with SIMV, so that when the patient takes a spontaneous breath, over and above the set frequency of mandatory volume-targeted breaths, inspiration is assisted to the set pressure support level.
Similar to CMV, in PCV the rate is fixed and cannot be increased by patient effort, but a difference is that it is the peak inflation pressure rather than the Vt that is set. Technically, the term for this mode is pressure-controlled continuous mandatory ventilation. On some ventilators it is also possible to deliver pressure-controlled A/C ventilation and pressure-controlled SIMV. Pressure-controlled inverse ratio ventilation (PCIRV) is not a separate mode, but rather PCV with the inspiratory phase longer than expiration. This variant of PCV has been used in patients who have severe hypoxemic respiratory failure in an attempt to improve oxygenation, but its popularity has waned because of the high incidence of hemodynamic compromise and barotrauma.
Fig 5: pressure support ventilation (PSV)
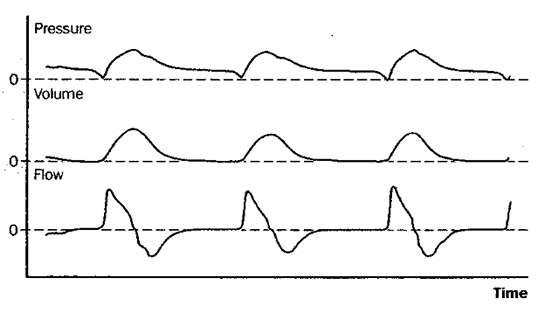
Figure 5: Schematic depiction of changes in pressure at the airway opening, lung volume, and flow during pressure support ventilation (PSV). Pressure support is essentially spontaneous breathing with a preset positive pressure boosting each inspiration. Patients on PSV receive no ventilation if apnea occurs.
Fig 6: pressure control ventilation (PCV)
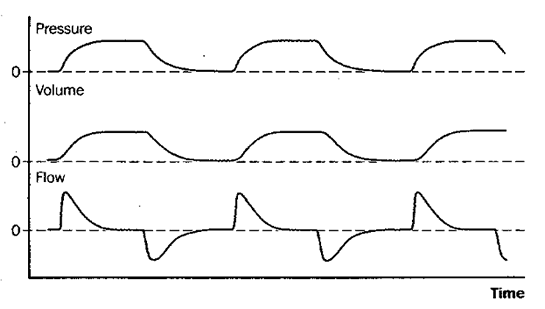
Figure 6: Schematic depiction of changes in pressure at the airway opening, lung volume, and flow during pressure control ventilation (PCV). Pressure control ventilation is analogous to controlled mechanical ventilation (CMV, Figure 2), in that the rate is fixed and the patient cannot trigger additional breaths or breathe spontaneously between mandatory breaths; it differs from CMV in that maximum inspiratory pressure rather than tidal volume is fixed, and both tidal volume and minute ventilation can vary if the patient’s lung-thorax compliance or airway resistance changes.
A key distinction between volume- and pressure-targeted modes relates to what happens when the mechanics of the patient-ventilator system change. When a patient who receives volume-targeted ventilation develops a pneumothorax or partial airway obstruction by inspissated secretions, the same Vt is delivered as before, but at higher peak and static airway pressures. However, with pressure-targeted ventilation, maximal airway pressure is preset and cannot increase under these circumstances. Instead, with obstruction in the airway or a decrease in compliance, the pressure stays the same and the delivered Vt decreases. Thus, the clinician needs to be aware that complications may be manifested differently in the different modes. When managing a patient whose pulmonary process may improve rapidly, as in acute asthma or pulmonary edema, the clinician needs to be prepared to make frequent ventilator adjustments when pressure ventilation is used.
Combined Modes
Most critical care ventilators of recent manufacture offer hybrid "modes" combining features of volume-targeted and pressure-targeted ventilation, in an attempt to avoid both the high peak airway pressures of volume ventilation and also the varying Vts that may occur with pressure ventilation. Manufacturers have attempted to make these combinations unique to their own machines in a competitive market, and as a result several apparently new modes have appeared on the scene. Essentially, these combinations consist either of volume ventilation with high inspiratory flow and a limitation on peak pressure, or of pressure ventilation regulated to provide a preset minute ventilation.
For example, recent Siemens/Maquet ventilator models offer pressure-regulated volume control (PRVC), in which all breaths are mandatory, the rate is fixed, and the inspiratory pressure is varied to maintain a preset Vt. These same ventilators also offer volume support, a combined mode that consists of PSV with a preset target Vt or minute volume, which the ventilator achieves by adding mandatory breaths, the inspiratory pressures of which are varied as necessary to achieve the set volume goal. Auto-mode, a third variation provided on recent Siemens/Maquet critical care ventilators, essentially combines PRVC and volume support. Auto-mode combines dual-control breath-to-breath time-cycled breaths with dual-control breath-to-breath flow-cycled breaths, patient effort or the lack thereof determining how individual breaths are cycled.
Each manufacturer features its own slightly-different blending of volume- and pressure-targeted modes. Other examples include auto-flow (Dräger), adaptive pressure regulation (Hamilton), and volume-assured pressure support (Bird). Automatic tube compensation (ATC), a feature of recent Dräger ventilators, is not a mode per se but rather an attempt to overcome resistive work of breathing added by the endotracheal tube. The operator enters the endotracheal tube diameter, and the ventilator applies positive pressure to overcome the desired proportion of calculated tube resistance via closed-loop control.
All these so-called combined modes are intended to give market advantage to the ventilator models that feature them. None has been demonstrated to have a detectable effect on clinical outcomes such as survival, complications, or weaning time.
In Table 5 [37k PDF*], a comparison is given of the most commonly used volume- and pressure-targeted ventilator modes in terms of their relative advantages and disadvantages.
Top of Page
Positive End-Expiratory Pressure (PEEP)
Manipulation of inspiration by means of the phase variables and modes just discussed is one of the two main processes involved in mechanical ventilation. The other is manipulation of end-expiratory pressure, which may be kept equal to that of the atmosphere or deliberately raised to produce positive end-expiratory pressure (PEEP). The application of PEEP has two primary purposes:
- To increase lung volume in patients who have acute lung restriction that produces hypoxemia; and,
- To reduce the effort required for patients to trigger the ventilator or breathe spontaneously in the presence of dynamic hyperinflation and auto-PEEP (see below).
When PEEP is applied to the breathing circuit connected to the closed respiratory system of an intubated patient, all breaths start and end at a pressure above ambient. Continuous pressurization of the system from which a patient breathes spontaneously is referred to as CPAP, a term applicable only during spontaneous breathing. Whenever positive pressure above the end-expiratory level is applied during inspiration, the term PEEP is used. Thus, a patient can be on A/C + PEEP, or on SIMV + CPAP, or on SIMV + PSV + PEEP.
As end-expiratory, end-inspiratory, and mean airway pressures are all increased in the presence of PEEP, the potential exists for a fall in cardiac output because of diminished venous return to the right side of the heart. Regional or generalized lung overdistention can also stretch pulmonary vessels, which reduces their caliber and increases pulmonary vascular resistance. In the presence of a reduced cardiac output secondary to either or both of these mechanisms, any gain in arterial oxygenation may be offset, and tissue oxygen delivery may actually fall. In addition, the application of PEEP may increase end-inspiratory lung volume to the point at which individual lung units become overdistended and rupture alveolar membranes, which leads to clinical barotrauma.
Unless PEEP is being adjusted according to the ARDS Network PEEP-FIO2 ladder according to institutional protocol, whenever feasible a systematic, incremental ‘PEEP trial’ should be performed in as controlled a manner as possible (Table 6 [32k PDF*]). Ideally, only one variable—the amount of PEEP—is altered during the trial, with Vt, fraction of inspired oxygen (FIO2 ), body position, and other factors that might affect oxygenation unchanged. An assessment for both favorable and adverse PEEP effects is made at each level as PEEP increased. As the condition of the patient may change over time, to determine the effects of PEEP most clearly the intervals at each level must be kept short.
As PEEP is increased, the occurrence cardiac impairment becomes progressively more likely. Direct measurement of cardiac output during the trial should be considered if any of the following circumstances exist:
- Levels of PEEP are to be used that are likely to impair cardiac function (e.g. 15 cm H2O or more);
- Unexplained tachycardia or other manifestations of possible hypovolemia are present; or
- The patient has underlying cardiac disease.
Changes in cardiac output and lung compliance are likely to occur rapidly following an increase in PEEP, and should be sought within the first 3-5 minutes at each level.
As PEEP is increased, PaCO2 is measured sequentially as the primary index of a favorable response. If a substantial increase in PaO2 occurs with no evidence of either cardiac impairment or alveolar overdistention (as assessed using static compliance), that PEEP level can be maintained and the FIO2 titrated downward to maintain the target PaCO2. Improvement in PaCO2 tends to occur more slowly than changes in cardiac function or compliance as PEEP is increased, and arterial blood gas specimens should be drawn 10 to 20 minutes after each change.
If PEEP is reduced prematurely, some alveoli may remain sufficiently unstable to collapse, which worsens oxygenation. If this happens, PEEP higher than the previous baseline level may be required to reopen the collapsed alveoli and, conceivably, the patient’s requirement for mechanical ventilation may be unnecessarily prolonged. It is thus important to be able to predict when patients are ‘ready’ for PEEP weaning. The protocol given in Table 7 [22k PDF*] is designed to facilitate PEEP withdrawal while protecting the patient from possible worsening of hypoxemia.
For patients whose arterial oxygenation has previously been shown to be ‘PEEP responsive’, the following criteria should be met before PEEP is reduced:
- Hemodynamic stability with no changes in PEEP during the previous 6–12 hours;
- No signs of sepsis present; and
- Adequate arterial oxygenation, as shown by a PaCO2 /FIO2 ratio of 200 mm Hg or more.
Using the protocol in Table 7 [22k PDF*], maximum protection from unintended hypoxemia caused by premature PEEP weaning can be achieved if a 3-minute trial of PEEP reduction is carried out.
Advances in understanding of ventilator-induced lung injury, along with results from major clinical trials, have led to a new overall approach to the ventilator management of acute lung injury (ALI) and the acute respiratory distress syndrome (ARDS), as discussed below. The lung-protective ventilation protocol summarized in Table 8 [43k PDF*] and the "FIO2 -PEEP Ladder" shown in Table 9 [20k PDF*] provide overall guidance for application of both PEEP trials and PEEP weaning as presented here.
Page 1 | go to Page 2
*Software capable of displaying a PDF is required for viewing or printing. You
may download a free copy of Adobe Reader from the Adobe
website.
Top of Page
|